In hindsight, it seems prophetic that the title of a Nature paper published on 1 March 1974 ended with a question mark: “Black hole explosions?” Stephen Hawking’s landmark idea about what is now known as Hawking radiation1 has just turned 50. The more physicists have tried to test his theory over the past half-century, the more questions have been raised — with profound consequences for how we view the workings of reality.
In essence, what Hawking, who died six years ago today, found is that black holes should not be truly black, because they constantly radiate a tiny amount of heat. That conclusion came from basic principles of quantum physics, which imply that even empty space is a far-from-uneventful place. Instead, space is filled with roiling quantum fields in which pairs of ‘virtual’ particles incessantly pop out of nowhere and, under normal conditions, annihilate each other almost instantaneously.
However, at an event horizon, the spherical surface that defines the boundary of a black hole, something different happens. An event horizon represents a gravitational point of no return that can be crossed only inward, and Hawking realized that there two virtual particles can become separated. One of them falls into the black hole, while the other radiates away, carrying some of the energy with it. As a result, the black hole loses a tiny bit of mass and shrinks — and shines.
Unexpected ramifications
The power of Hawking’s 1974 paper lies in how it combined basic principles from the two pillars of modern physics. The first, Albert Einstein’s general theory of relativity — in which black holes manifest themselves — links gravity to the shape of space and time, and is typically relevant only at large scales. The second, quantum physics, tends to show up in microscopic situations. The two theories seem to be mathematically incompatible, and physicists have long struggled to find ways to reconcile them. Hawking showed that the event horizon of a black hole is a rare place where both theories must play a part, with calculable consequences.
Science mourns Stephen Hawking’s death
And profoundly unsettling ones at that, as quickly became apparent. The random nature of Hawking radiation means that it carries no information whatsoever. As Hawking soon realized2, this means that black holes slowly erase any information about anything that falls in, both when the black hole originally forms and subsequently as it grows — in apparent contradiction to the laws of quantum mechanics, which say that information can never be destroyed. This conundrum became known as the black-hole information paradox.
It has since turned out that black holes should not be the only things that produce Hawking radiation. Any observer accelerating through space could, in principle, pick up similar radiation from empty space3. And other analogues of black-hole shine abound in nature. For example, physicists have shown that in a moving medium, sound waves trying to move upstream seem to behave just as Hawking predicted. Some researchers hope that these experiments could provide hints as to how to solve the paradox.
A scientific wager
In the 1990s, the black-hole information paradox became the subject of a celebrated bet. Hawking, together with Kip Thorne at the California Institute of Technology (Caltech) in Pasadena, proposed that quantum mechanics would ultimately need to be amended to take Hawking radiation into account. Another Caltech theoretical physicist, John Preskill, maintained that information would be found to somehow be preserved, and that quantum mechanics would be saved.
But in 1997, theoretical physicist Juan Maldacena, who is now at the Institute for Advanced Study in Princeton , New Jersey, came up with an idea that indicated Hawking and Thorne might be wrong4. His paper now has more than 24,000 citations, even more than the 7,000 or so times Hawking’s paper has been cited. Maldacena suggested that the Universe — including the black holes it contains — is a type of hologram, a higher-dimensional projection of events that occur on a flat surface. Everything that happens on the flat world can be described by pure quantum mechanics, and so preserves information.
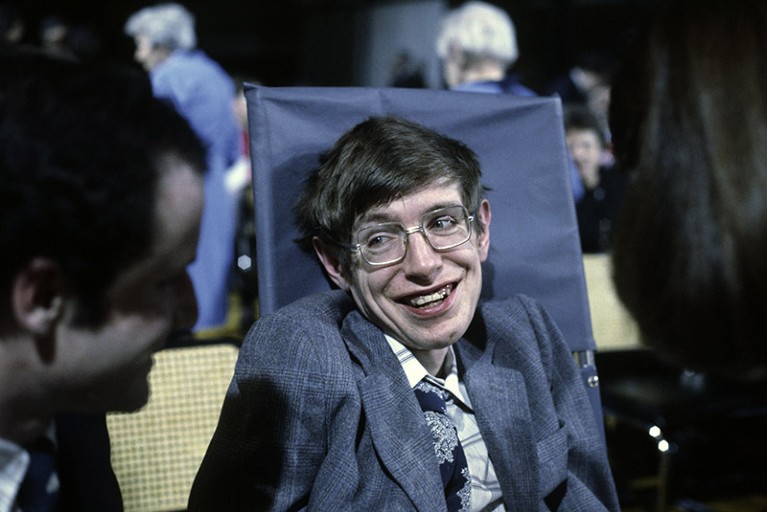
Stephen Hawking worked on the black hole information paradox throughout his life.Credit: Santi Visalli/Getty
At face value, Maldacena’s theory doesn’t fully apply to the type of Universe that we inhabit. Moreover, it did not explain how information could escape destruction in a black hole — only that it should, somehow. “We don’t have a concrete grasp of the mechanism,” says Preskill. Physicists, including Hawking, have proposed countless escape mechanisms, none of which has been completely convincing, according to Preskill. “Here it is, 50 years after that great paper, and we’re still puzzled,” he says. (Maldacena’s ideas were enough to change Hawking’s mind, however, and he conceded the bet in 2004.)
A quantum conundrum
Attempts to solve the information paradox have grown into a thriving industry. One of the ideas that has gained traction is that each particle that falls into a black hole is linked to one that stays outside through quantum entanglement — the ability of objects to share a single quantum state even when far apart. This connection could manifest itself in the geometry of space-time as a ‘wormhole’ joining the inside of the event horizon with the outside.
Entanglement is also one of the crucial features that make quantum computers potentially more powerful than classical ones. Moreover, in the past decade, the link between black holes and information theory has become only stronger, as Preskill and others have investigated similarities between what happens in holographic projections and the types of error-correction algorithm developed for quantum computers. Error correction is a way of storing redundant information that enables a computer — whether classical or quantum — to restore corrupted bits of information. Some researchers see quantum computation theory as the key to solving Hawking’s paradox. When creating a black hole, the Universe could be similarly storing several versions of its information — some inside the event horizon, some outside — so that the destruction of the black hole does not erase any history.
Hawking’s latest black-hole paper splits physicists
But other researchers think that the full resolution of the information paradox might have to wait until another big problem is solved — that of reconciling gravity with quantum physics. Hawking continued working on the problem almost up until his death, but with no clear outcome.
As for the title of Hawking’s paper, seeing actual black-hole explosions is a possibility that astronomers take seriously. Large black holes act like very cold bodies, but smaller ones are hotter, which makes them shrink faster; and the particles they shed should become more and more energetic, reaching a culmination when the black hole disappears. Hawking showed that ‘ordinary’ stellar-mass black holes, which form when massive stars collapse in on themselves at the end of their lives, take many times longer than the age of the Universe to get to this point. But, in principle, black holes with a range of smaller masses could have formed from random fluctuations in the density of matter during the first moments after the Big Bang. If a primordial black hole of the right mass were to fizzle into non-existence somewhere near the Solar System, it could be picked up by neutrino and γ-ray observatories.
Astronomers have not seen any black holes explode so far, but they are still on the lookout5. Such an observation would have certainly earned Hawking the Nobel Prize that eluded him all his life. As it is, the questions produced by his simple, inquisitive paper title look set to nourish the intersection between cosmology and physics for a good few years yet.